Synth Bio
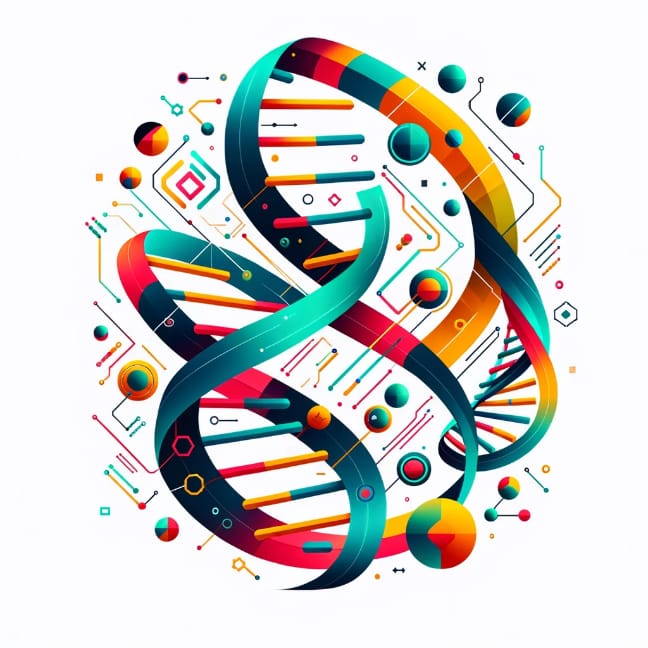
Synthetic biology (synth bio) is an interdisciplinary field combining biology and engineering. Its goal is to design and construct new biological parts, devices, and systems not found in nature, or to redesign existing biological systems for useful purposes. It applies engineering principles to biology, enabling the creation of new biological systems or the modification of existing ones.
As a pioneering field at the forefront of technological innovation synth bio has a wide range of applications that extend from genetic engineering and the creation of novel biomaterials to biofuel production and the development of synthetic organisms for medical, agricultural, and environmental sustainability. It is rapidly advancing, continually harnessing the latest advancements in genomics and bioinformatics.
Synth bio will shape the future in profound ways. For instance, it will be instrumental in transforming physical products into digital services enabling the "digitization of matter." In this emerging paradigm, consumers might purchase source code, then use tools like DNA synthesizers and 3D printers to materialize products.
DNA synthesizers enable the fabrication of custom genetic materials, leading to bespoke biological products. Similarly, 3D printers convert digital code into physical objects by layering material, such as plastic, metal, or resin. This integration of technology represents a paradigm shift, where physical products are no longer merely traded in conventional ways, but rather, are accessible online and produced on-site and on-demand. When DNA synthesizers and 3D printers become widely adopted, they will lead to lower marginal costs in both the production and distribution of products.
As someone without an academic background in biology, I've been eager to figure out how to deepen my understanding of synth bio, so I've turned to several books on the subject. I began my exploration with a series of accessible lectures called "Synthetic Biology: Life’s Extraordinary New Worlds" by Milton Muldrow Jr., PhD, and I have recently started reading "The Genesis Machine" by Amy Webb and Andrew Hessel.
Some of the topics I've enjoyed learning about:
- DNA sequencers are instruments used to determine the precise order of nucleotides within a DNA molecule. Understanding the sequence of DNA is crucial for a wide range of biological and medical research. DNA sequencers have revolutionized biological sciences, enabling detailed studies of genetics and driving advancements in medicine, agriculture, and biotechnology.
- DNA sequencers, adapted to work with RNA through a process called reverse transcription, were pivotal in decoding the SARS-CoV-2 virus. In this process, the virus's RNA was converted into complementary DNA using an enzyme called reverse transcriptase. This is necessary because RNA and DNA, while structurally similar, have key differences such as the sugar in their backbone and the use of uracil in RNA instead of thymine in DNA. Once converted, the genetic sequence was analyzed using standard DNA sequencing techniques. This allowed scientists to quickly identify the virus, understand its genetic structure, and expedite the development of diagnostic tests and vaccines.
- DNA synthesis refers to the process of artificially creating strands of DNA in the laboratory. DNA synthesizers are instruments used to chemically construct DNA molecules.
- After the SARS-CoV-2 virus was genetically sequenced, DNA synthesizers were used to create segments of the virus's genome for research purposes.
- Since the virus is RNA-based, DNA synthesizers were used to create DNA sequences complementary to the RNA genome of the virus.
- The synthesized DNA was then used as a template to produce RNA segments that correspond to parts of the viral genome. This RNA was used to study the virus's properties, mechanisms of infection, and immune response it elicited in the body.
- This allowed for a remarkably quick response from the scientific community, leading to the unprecedented rapid development of effective vaccines and treatments.
- Metabolic engineering is a scientific field focused on altering metabolic pathways in organisms, aiming to boost the production of specific substances or to imbue these organisms with novel capabilities. Commonly utilized in the production of pharmaceuticals, biofuels, and various materials via microbial systems, this discipline is pivotal for operations involving genetically engineered yeast or bacterial cells. In tandem with bioreactors, metabolic engineering significantly improves the efficiency and output of bioproduction processes.
- I've been inspired by how the company Perfect Day applies metabolic engineering to produce dairy proteins from plant sugars at scale.
- Directed evolution is a technique in molecular biology and bioengineering that simulates the natural selection process in a laboratory setting, guiding the evolution of proteins or nucleic acids towards specific objectives set by researchers. This method significantly speeds up the evolutionary process that naturally takes millennia, enabling the rapid evolution of biomolecules in the lab. As a result, it has emerged as an influential tool for the engineering of biomolecules across various applications. Particularly effective when our grasp of biological systems is incomplete, directed evolution leverages nature's own mechanisms to steer these modifications to achieve desired results.
- Increasingly, directed evolution, CRISPR, and biological modification approaches such as metabolic engineering are being used together to evolve organisms or biomolecules towards specific desired outcomes. 🤯
- DNA computing uses DNA, along with biochemistry and molecular biology tools, to store and process data, diverging significantly from traditional electronic computing. Its standout feature is the remarkable data storage capacity of DNA, with a single gram capable of holding approximately 215 petabytes (215 million gigabytes) of data. Beyond storage, DNA strands can be specifically designed and synthesized to perform logic operations, essential for computing tasks. This capability is advantageous for problems that benefit from massive parallel processing, and it's more energy-efficient compared to traditional computing methods.
- Transcriptors act as the biological equivalent of an electronic transistor, but they operate using DNA and RNA to carry out logic gate operations, key in computing for decision-making processes. Fundamental computer components—data storage, information transfer, and basic logic—can theoretically be replicated with biological substances. Transcriptors highlight the capability of DNA and RNA to imitate conventional computing components, reinforcing the significance of biological substances in the ongoing advancement of computing technologies.
- Gene drives are a genetic technology that alters the typical rules of inheritance, ensuring a specific gene is passed down at a higher rate than the usual 50% in sexual reproduction. This is achieved through gene editing techniques like CRISPR, which insert a gene drive into an organism’s DNA so that it is copied into both chromosomes of a pair. This ensures almost all offspring inherit the engineered gene. Gene drives have potential uses in public health, agriculture, and conservation, such as controlling disease vectors or invasive species. However, they pose significant ecological and ethical concerns, including the risk of unintended consequences on ecosystems, which necessitates careful consideration and regulation. Gene drive reversal involves the use of additional gene editing techniques to either neutralize or overwrite the effects of the original gene drive. It can be achieved by introducing a second gene drive designed to counteract the first one, or by using methods that specifically target and inactivate the components of the original gene drive. The concept of reversibility is important in the development and ethical deployment of gene drives, as it provides a potential way to mitigate unintended ecological effects or other risks associated with their use. ðŸ«
As I continue to delve deeper into synth bio, the convergence of biology with technology not only fascinates me but also reinforces my optimism about the ongoing transformative impact of technology on our collective future.